Genomics England
Posted on November 10, 2021
Key message
Incredible — findings from UK’s 100,000 Genomes Pilot show that whole genome sequencing
- helped make genetic diagnoses in 25% of respondents,
- 14% of whom may not have been diagnosed otherwise.
A testament to the power of genomic sequencing in healthcare.
by Trevor Mundel, President of Global Health, Bill and Melinda Gates Foundation
A world-first scientific study, published today in the New England Journal of Medicine, has shown that whole genome sequencing (WGS) can uncover new diagnoses for people across the broadest range of rare diseases investigated to date and could deliver enormous benefits across the NHS.
The pilot study of rare undiagnosed diseases involved analysing the genes of 4,660 people from 2,183 families — all of whom were early participants in the 100,000 Genomes Project. The ground-breaking Project, led by Genomics England and NHS England, was established in 2013 to sequence 100,000 whole genomes from NHS patients and their families.
The pilot study, led by Genomics England and Queen Mary University of London and undertaken in partnership with the National Institute for Health Research (NIHR) BioResource, found that using WGS led to a new diagnosis for 25% of the participants. Of these new diagnoses, 14% were found in regions of the genome that would be missed by other conventional methods, including other types of non-whole genomic tests.
Many of the participants had gone through years of appointments, without getting any answers. By having their whole genome sequenced diagnoses were uncovered that would not have previously been detectable.
The pilot study shows that WGS can effectively secure a diagnosis for patients, save the NHS vital resources and pave the way for other interventions.
Participants who received a diagnosis through the pilot include:
- a 10-year-old girl whose previous seven-year search for a diagnosis had multiple intensive care admissions over 307 hospital visits at a cost of £356,571. Genomic diagnosis enabled her to receive a curative bone marrow transplant (at a cost of £70,000). In addition, predictive testing of her siblings showed no further family members were at risk.
- a man in his 60s who had endured years of treatment for a serious kidney disease, including two kidney transplants. Already knowing his daughter had inherited the same condition, a genomic diagnosis made by looking at the whole genome for him and his daughter enabled his 15-year-old granddaughter to be tested. This revealed she had not inherited the disease and could cease regular costly check-ups.
- a baby who became severely ill immediately after birth and sadly died at four months but with no diagnosis and healthcare costs of £80,000. Analysis of his whole genome uncovered a severe metabolic disorder due to inability to take vitamin B12 inside cells explaining his illness. This enabled a predictive test to be offered to his younger brother within one week of his birth. The younger child was diagnosed with the same disorder but was able to be treated with weekly vitamin B12 injections to prevent progression of the illness.
For around a quarter of study participants, their diagnosis meant they were able to receive more focused clinical care. This included further family screening, dietary change, provision of vitamins and / or minerals and other therapies.
The study is the first to analyse the diagnostic and clinical impact of WGS for a broad range of rare diseases within a national healthcare system. The findings support its widespread adoption in health systems worldwide.
The high performance of WGS for specific conditions observed in the pilot study — including intellectual disability, vision and hearing disorders of 40–55% diagnostic yield — has underpinned the case for the inclusion of WGS to diagnose specific rare diseases as part of the new NHS National Genomic Test Directory.
The pilot study was also conducted in partnership with the National Institute for Health Research (NIHR) and Illumina who undertook the sequencing, and it was funded by the NIHR, the Wellcome Trust, the Medical Research Council, Cancer Research UK, the Department of Health and Social Care, and NHS England.
ORIGINAL PUBLICATION
Please, find below, an excerpt of the original publication, edited by the Editor of the Blog.
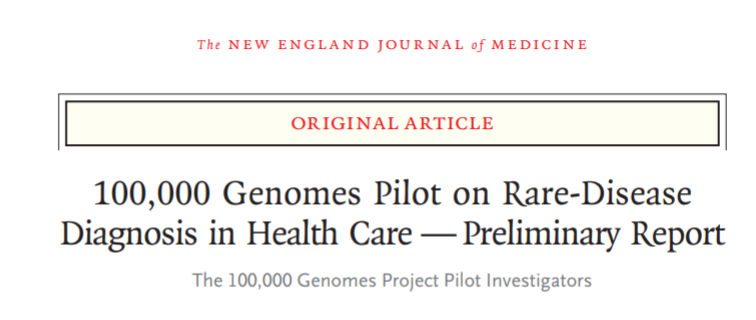
100,000 Genomes Pilot on Rare-Disease Diagnosis in Health Care — Preliminary Report
New England Journal of Medicine
List of authors. The 100,000 Genomes Project Pilot Investigators (see the original publication)
Drs. Smedley and Smith, Mr. Martin, and Drs. E.A. Thomas, McDonagh, Cipriani, Ellingford, Arno, Tucci, Vandrovcova, Chan, and H.J. Williams and Drs. Scott, Fowler, Rendon, and Caulfield contributed equally to this article.
November 11, 2021
newsmedical
Abstract
BACKGROUND
The U.K. 100,000 Genomes Project is in the process of investigating the role of genome sequencing in patients with undiagnosed rare diseases after usual care and the alignment of this research with health care implementation in the U.K. National Health Service.
Other parts of this project focus on patients with cancer and infection.
METHODS
We conducted a pilot study involving 4660 participants from 2183 families, among whom 161 disorders covering a broad spectrum of rare diseases were present.
We collected data on clinical features with the use of Human Phenotype Ontology terms, undertook genome sequencing, applied automated variant prioritization on the basis of applied virtual gene panels and phenotypes, and identified novel pathogenic variants through research analysis.
RESULTS
Diagnostic yields varied among family structures and were highest in family trios (both parents and a proband) and families with larger pedigrees.
Diagnostic yields were much higher for disorders likely to have a monogenic cause (35%) than for disorders likely to have a complex cause (11%).
Diagnostic yields for intellectual disability, hearing disorders, and vision disorders ranged from 40 to 55%. We made genetic diagnoses in 25% of the probands.
A total of 14% of the diagnoses were made by means of the combination of research and automated approaches, which was critical for cases in which we found etiologic noncoding, structural, and mitochondrial genome variants and coding variants poorly covered by exome sequencing.
Cohortwide burden testing across 57,000 genomes enabled the discovery of three new disease genes and 19 new associations.
Of the genetic diagnoses that we made, 25% had immediate ramifications for clinical decision making for the patients or their relatives.
CONCLUSIONS
Our pilot study of genome sequencing in a national health care system showed an increase in diagnostic yield across a range of rare diseases. (Funded by the National Institute for Health Research and others.)
The 100,000 Genomes Pilot on Rare-Disease Diagnosis
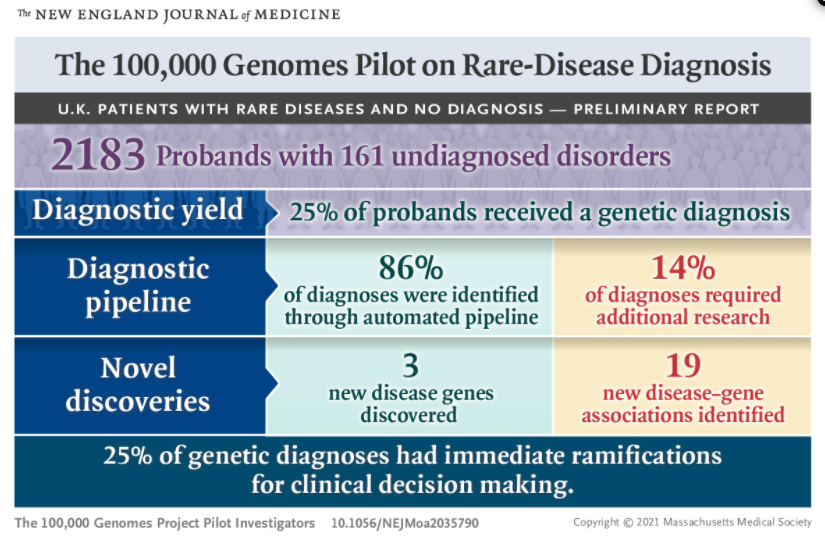
100,000 Genomes Pilot on Rare-Disease Diagnosis in Health Care — Preliminary Reportv (excerpted below)
Introduction
Rare diseases are a worldwide health care challenge, with approximately 10,000 disorders affecting 6% of the population in Western societies.1,2
More than 80% of rare diseases have a genetic component, and these conditions are disabling and expensive to manage. One third of children with a rare disease die before their fifth birthday.1
The adoption of next-generation sequencing has improved rates of diagnosis of rare diseases over the past decade.3–5
However, the majority of patients with rare diseases remain without a molecular diagnosis after standard diagnostic testing.3–5
To address this lack of diagnosis, the U.K. government launched the 100,000 Genomes Project in 2013 to apply whole-genome sequencing to the study of rare diseases, cancers, and infections in a national health care setting.6
To assess the effect of the whole-genome–sequencing approach on the genetic diagnosis of rare diseases in the National Health Service (NHS) in the United Kingdom, we carried out a pilot study in which we enrolled families and undertook detailed clinical phenotyping of the proband.4
We collected electronic health records from all participants and stored these together with the genomic and clinical data in a computer environment with multi-petabytes of storage (the Genomics England research environment).5 When necessary, we validated diagnostic variants in the laboratory and performed computational analyses.
Methods
See the original publication
Results
See the original publication
Discussion
Our findings show a substantial increase in yield of genomic diagnoses made in patients with the use of genome sequencing across a broad spectrum of rare disease. The enhanced diagnostic benefit was observed regardless of whether participants had undergone previous genetic testing (diagnostic yields were 31% among those who had undergone testing and 33% among those who had not). In 25% of those who received a genetic diagnosis, there was immediate clinical actionability. The standardization of procedures — from the enrollment of patients to the return of NHS-validated results to clinicians — was critical to our success. For example, the collection of clinical data with the use of disease-specific data models and HPO terms enabled diagnoses, which confirmed the value of standardization with the use of ontology terms and clinical annotation in precision medicine.31 These additional diagnoses, beyond the 264 (49% of total diagnoses) observed with the use of the single-disease virtual panel, came from the use of Exomiser and additional applied panels. The diagnostic discoveries derived by combining research, decision support, and clinical validation and assessment leveraged an additional 72 diagnoses.
Diagnostic yield was influenced by family structure, and for disorders likely to have mendelian inheritance and a single-gene etiologic factor, our yield increased to 35%: ophthalmologic, metabolic, and neurologic disorders yielded the greatest percentage of diagnoses. The scale of our data set enabled cohortwide burden testing, which identified numerous novel disease–gene associations, including three that have now been confirmed and 19 with compelling evidence that are likely to be confirmed in independent data sets.
Of the diseases we diagnosed with the use of genome sequencing, 13% were caused by mutations in noncoding sequence or mitochondrial genomes, tandem repeat expansions in persons with Huntington’s disease, and a wide range of structural variants with nucleotide resolution of breakpoints (which were identified with the use of a new random forest method). An additional 2% of the diagnoses involved coding variants in regions of low coverage on exome sequencing. Our results provide new evidence of the value of genome sequencing and mirror the findings in a previous study in which 53% of the participants who received new diagnoses from genome sequencing had previously undergone exome sequencing.5
Previous studies have shown how next-generation sequencing can lead to diagnoses, with yields of 25 to 29% with the use of exome sequencing in persons who had received no previous genetic testing.32–34 The Undiagnosed Disease Network reported a diagnostic yield of 26% with the use of a mixture of whole-exome and whole-genome sequence analysis in 382 patients,5 and another study of genome sequencing showed a yield of 42% among 50 probands with intellectual disability who had previously undergone testing.35 Among probands with a broad range of disorders (161 in total) with an unmet diagnostic need, we obtained results that were similar to those in the previous studies. Our approach is limited to diagnoses that are readily made by means of short-read genome sequencing. Fully phased, long-read sequencing better detects structural variation and delivers sequence information from parts of the genome that are poorly captured by short-read sequencing.36
The findings from our pilot study support the case for genome sequencing in the diagnosis of certain specific rare diseases in the new NHS National Genomic Test Directory.37 In patients with specific disorders, such as intellectual disability, genome sequencing is now the first-line test in the NHS (Table S12). With a new National Genomic Medicine Service, the NHS in England is in the process of sequencing 500,000 whole genomes in rare disease and cancer in health care. We hope that our findings will assist other health systems in considering the role of genome sequencing in the care of patients with rare diseases.
References
See original publication
Support
Supported by the NIHR, the Wellcome Trust, the Medical Research Council (MRC), Cancer Research U.K., the Department of Health and Social Care, and NHS England. The NIHR BioResource is funded by the NIHR. Drs. Caulfield and Ouwehand are NIHR senior investigators. Dr. Chinnery is a Wellcome Trust Principal Research Fellow (212219/Z/18/Z) and an NIHR Senior Investigator who receives support from the MRC Mitochondrial Biology Unit (MC_UU_00015/9), the MRC International Centre for Genomic Medicine in Neuromuscular Disease (MR/S005021/1), and the NIHR Biomedical Research Centre (BRC). Dr. Wedderburn’s work is supported by grants from Versus Arthritis (21593), the NIHR BRC at Great Ormond Street Hospital, and the MRC (MR/R013926/1). Drs. Smedley, Cacheiro, and Cipriani receive support from the National Institutes of Health (NIH, grant 5-UM1-HG006370). Dr. Smedley’s team that performed much of the analysis was supported by grants from the NIH (1R24OD011883, U54 HG006370, and 1R01HD103805–01). Dr. Arno’s work is supported by a Fight for Sight (United Kingdom) Early Career Investigator Award (5045/46), NIHR BRC at Great Ormond Street Hospital Institute for Child Health, and Moorfields Eye Charity (Stephen and Elizabeth Archer in memory of Marion Woods). The Moorfields–University College London (UCL) Institute of Ophthalmology team is additionally funded by NIHR BRC at Moorfields Eye Hospital and UCL Institute of Ophthalmology.
Disclosure forms provided by the authors are available with the full text of this article at NEJM.org.
Drs. Smedley and Smith, Mr. Martin, and Drs. E.A. Thomas, McDonagh, Cipriani, Ellingford, Arno, Tucci, Vandrovcova, Chan, and H.J. Williams and Drs. Scott, Fowler, Rendon, and Caulfield contributed equally to this article.
The views expressed are those of the authors and not necessarily those of the National Health Service (NHS), the National Institute for Health Research (NIHR), or the Department of Health and Social Care.
We thank the personnel at NIHR BioResource for their partnership in this study; all the health care teams at Addenbrooke’s Hospital in Cambridge, Great Ormond Street Hospital NHS Foundation Trust, University College London NHS Foundation Trust, Guy’s and St. Thomas’ Hospital, Barts Health, Oxford University Hospitals NHS Foundation Trust, Manchester University NHS Foundation Trust, and the Newcastle Hospitals NHS Foundation Trust; the NHS patients and their families who made this work possible; all those across the world who have contributed to the PanelApp knowledge base and to the validation and reporting working group (Dr. Dom McMullan, Dr. Helen Firth, Dr. Steve Abbs, and Dr. Sian Ellard) for their role in supporting the development of the bioinformatics pipeline and reporting process; Dr. David Bick and Dr. Gil McVean for providing feedback on our work; Dr. Dame Sue Hill and the team at NHS England for the work to fund and establish the 13 GMCs, which enabled the NHS contribution that included the clinical return of results within the NHS in a standardized and validated format that led to the confirmation of the diagnoses, provided additional information, and led to the patient benefit reported; the Illumina Laboratory Services team at Hinxton for genome sequencing and secondary analysis; and the developers of the Human Phenotype Ontology (Monarch Initiative) and Exomiser (funded by the NIH Office of the Director [1R24OD011883]) for the support that was provided through these resources.
Author Affiliations
Dr. Caulfield can be contacted at m.j.caulfield@qmul.ac.uk or at Genomics England, William Harvey Research Institute, Queen Mary University of London, London EC1M 6BQ, United Kingdom.
The authors’ full names, academic degrees, and affiliations are listed in the Appendix.
About the authors & affiliations (excerpted — only first names of the list)
For the complete list of authors, please, refer to the original publication.
The authors’ full names and academic degrees are as follows:
Damian Smedley, Ph.D., Katherine R. Smith, Ph.D., Antonio Martin, M.Sc., Ellen A. Thomas, M.D., Ellen M. McDonagh, Ph.D., Valentina Cipriani, Ph.D., Jamie M. Ellingford, Ph.D., Gavin Arno, Ph.D., Arianna Tucci, M.D., Jana Vandrovcova, Ph.D., Georgia Chan, Ph.D., Hywel J. Williams, Ph.D., e all
Selected images
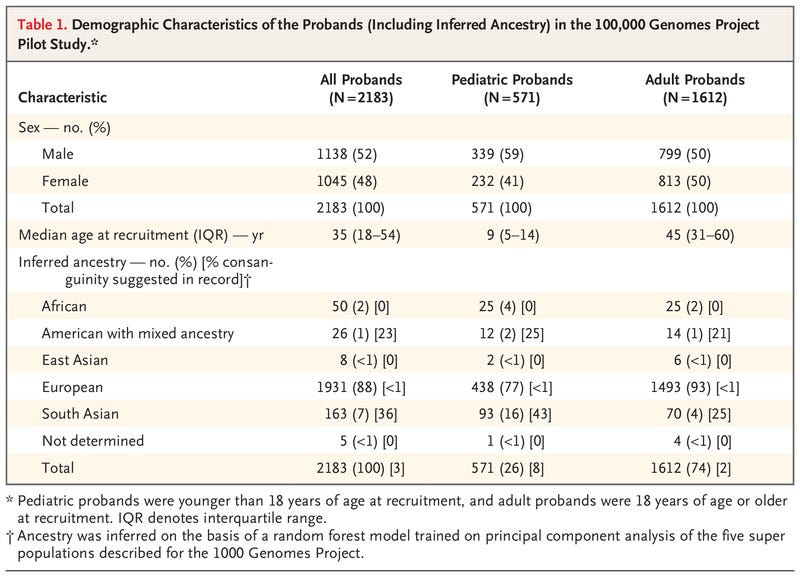
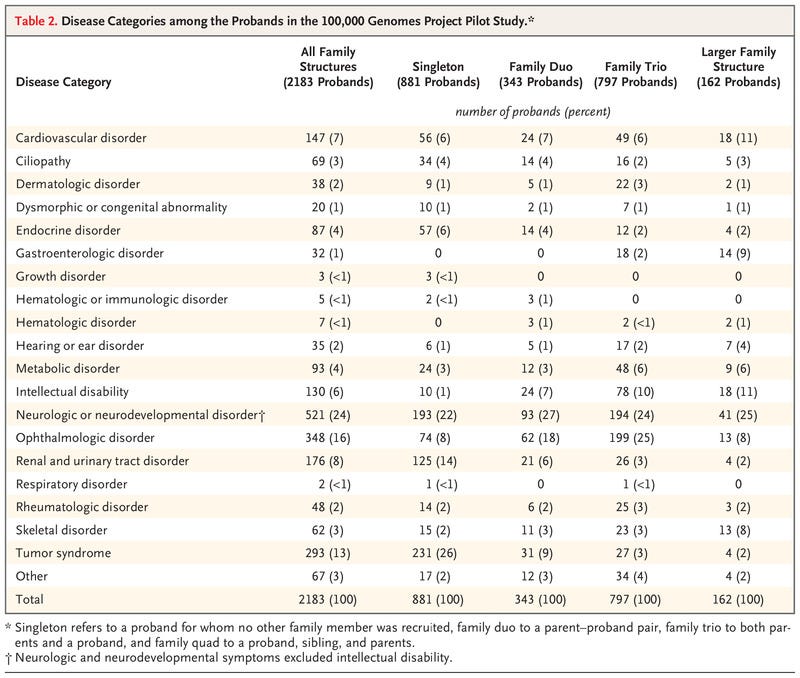
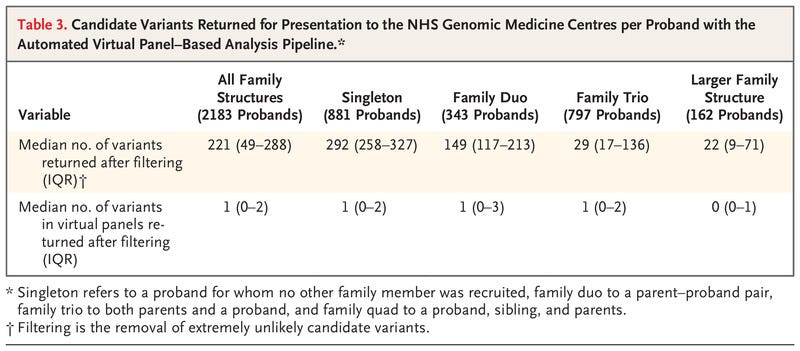
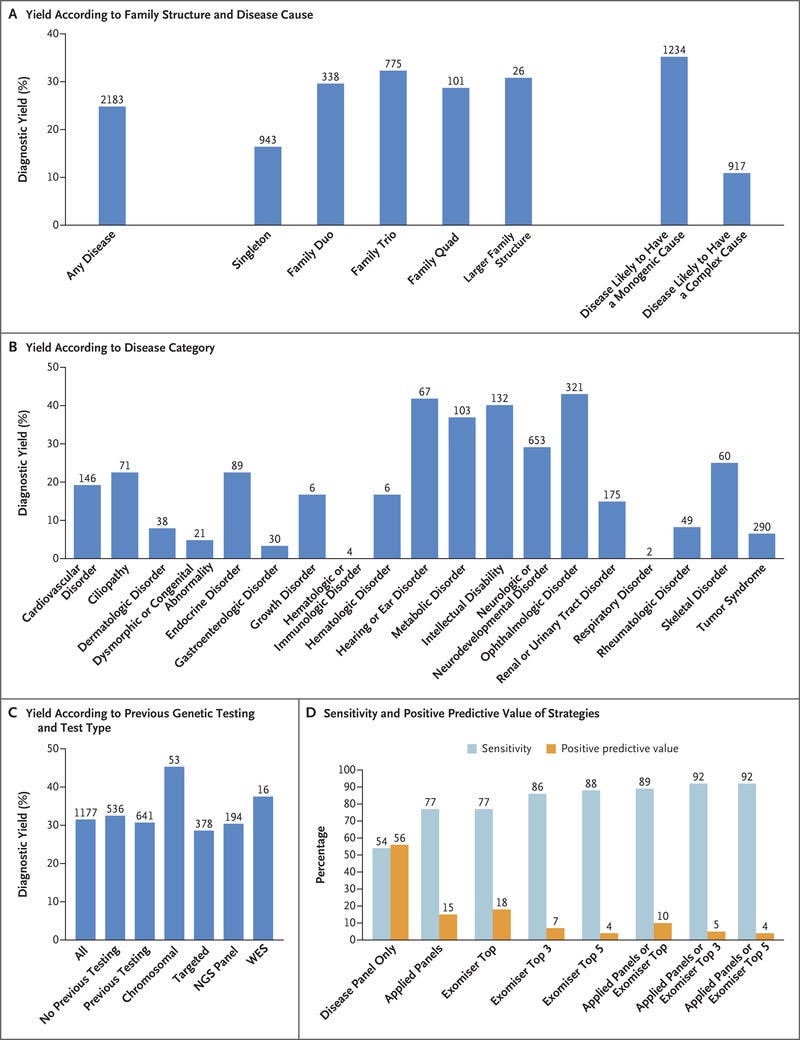
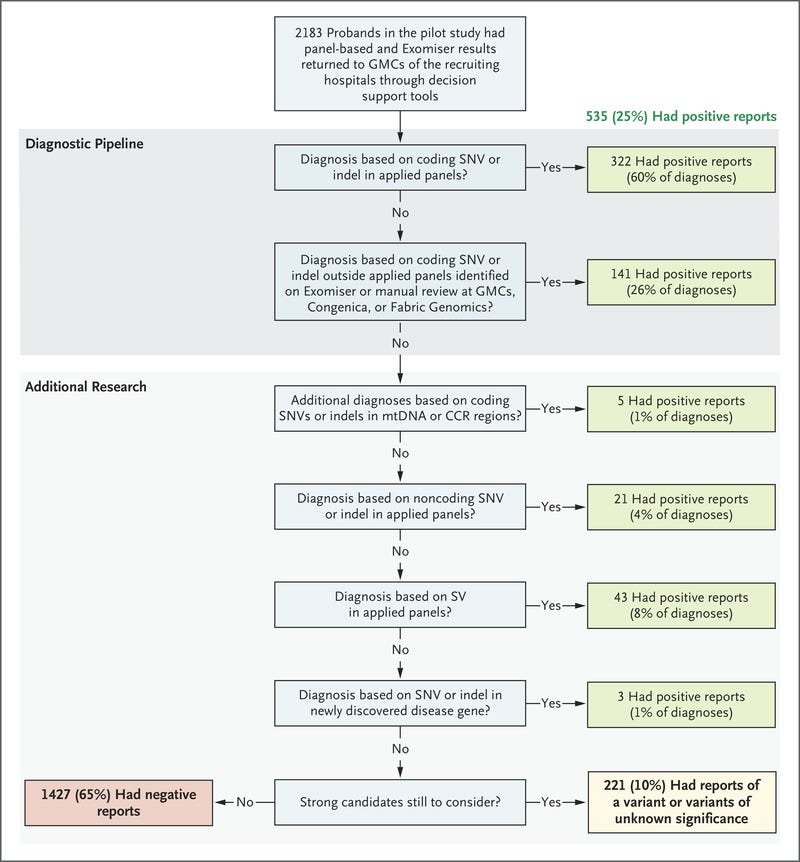
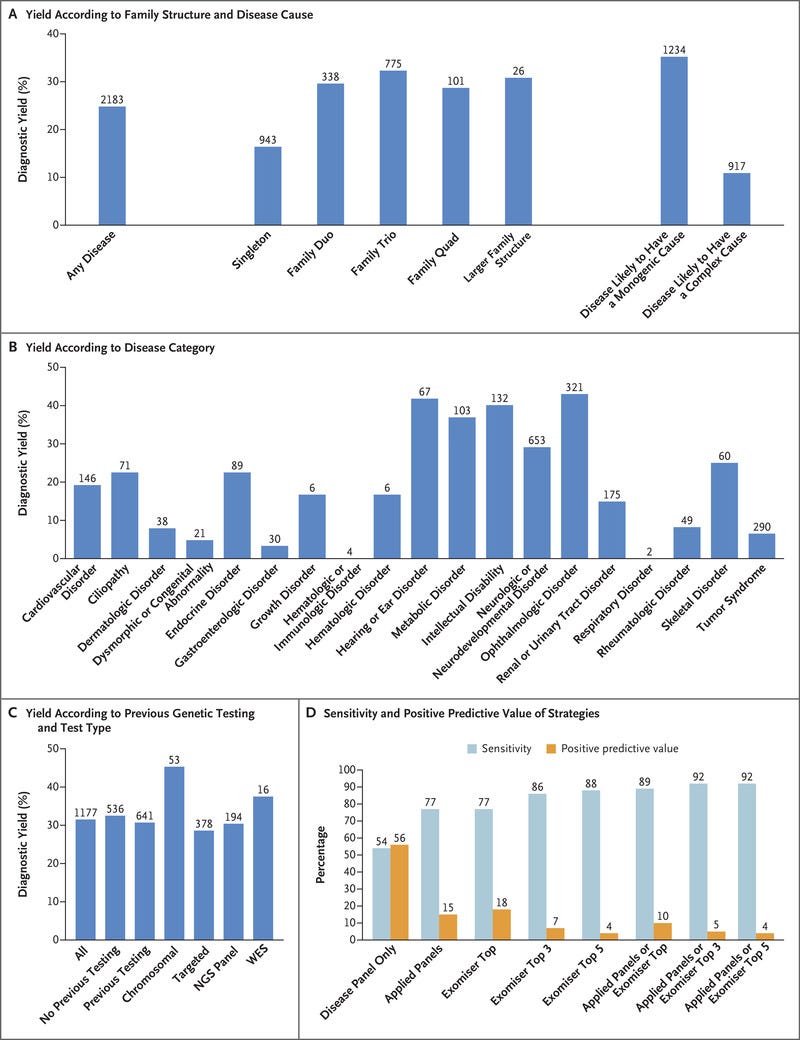
Originally published at https://www.nejm.org.
TAGS: Genomic Testing, Rare Diseases, Atlas of Diseases